Gravitational waves research
We develop new technologies for the next generation European gravitational-wave detector Einstein Telescope, designed to observe the whole Universe in GW spectrum. Gravitational waves are ripples on the fabric of space-time born in catastrophic collisions of the most massive and densest objects in the universe, the black holes and neutron stars.
To detect these tiny ripples, Einstein Telescope, the Europe’s next generation GW observatory will be built, and our group is working on a range of advanced technologies to make this reality. Maastricht University is a home for the unique experimental facility, the ET Pathfinder, that will become a testbed for a range of groundbreaking cryogenic and quantum technologies to be used in the ET.
- Precision Interferometry
- General Relativity and GW sources
- GW data analysis
- Quantum noise and quantum metrology
- Optical absorption and optical coating design
- Cryogenics and vacuum technology
Gravitational-waves detectors
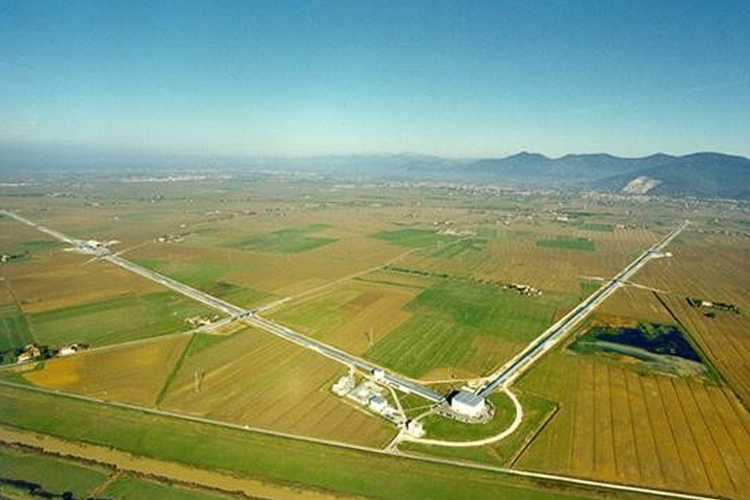
All currently operating laser gravitational-wave detectors follow the same measurement principle based on an advanced version of a Michelson interferometer: light from a stable laser source is split into two orthogonal beams that travel inside vacuum tubes that are several kilometres long. Highly efficient mirrors at the end of the tubes send the light back, where the light waves overlap (interfere, hence interferometer) and create a distinctive bright and dark pattern. This pattern depends on the difference in light travel time along the two arms, and therefore any changes in the arm length caused by a passing gravitational wave get converted in a fluctuating brightness signal at the interferometer output.
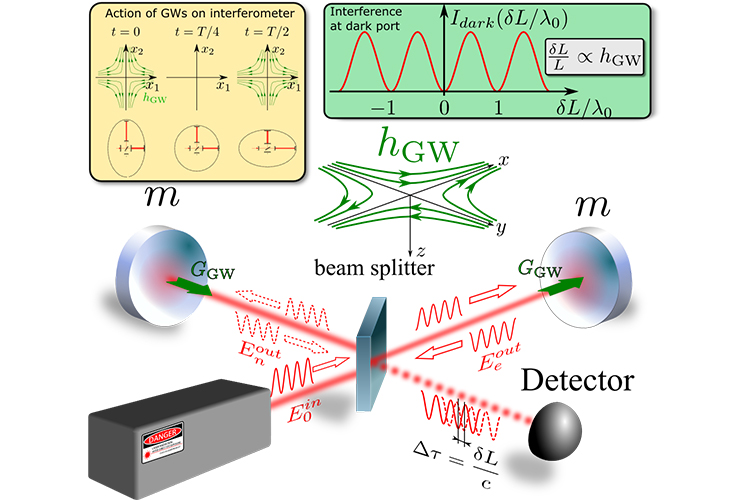
A plethora of other effects also cause the arm lengths to fluctuate and might hide potential gravitational-wave sources: these effects add noise to the gravitational-wave signal and so a key part in developing sensitive gravitational-wave detectors is to identify and reduce noise sources. Over the past decades, scientists have been relentless in this endeavor and with the detection of the first gravitational-wave signal GW150914 in 2015, their efforts have paid off.
Nowadays, the sensitivity of the operating gravitational-wave detectors is so high that the Brownian motion (thermal fluctuations) of the interferometer mirrors becomes limiting. The current detectors, such as Advanced LIGO and Advanced Virgo, operate with mirrors at room temperature. Cooling the test masses to cryogenic temperatures could reduce the thermal noise, but cryo-coolers, just like household fridges, produce quite a bit of noise themselves from their compressors. In addition, this approach does not work with the current mirror material fused silica, as it loses its excellent mechanical properties at low temperatures. Designs for the next generation of gravitational-wave detectors, such as the Einstein Telescope (ET) and Cosmic Explorer (CE) instead plan to use mirrors made from silicon. While silicon is a well-known material in the semiconductor industry, new techniques have to be developed and tested to use it for mirrors in gravitational-wave detectors, to keep these mirrors cold without adding too much noise, and even to bounce light back and forth between them: silicon is not transparent for the near-infrared lasers that the current detectors use, so new laser sources further into the infrared (around 1550nm to 2100nm) need to be developed and tested as well.
ETpathfinder
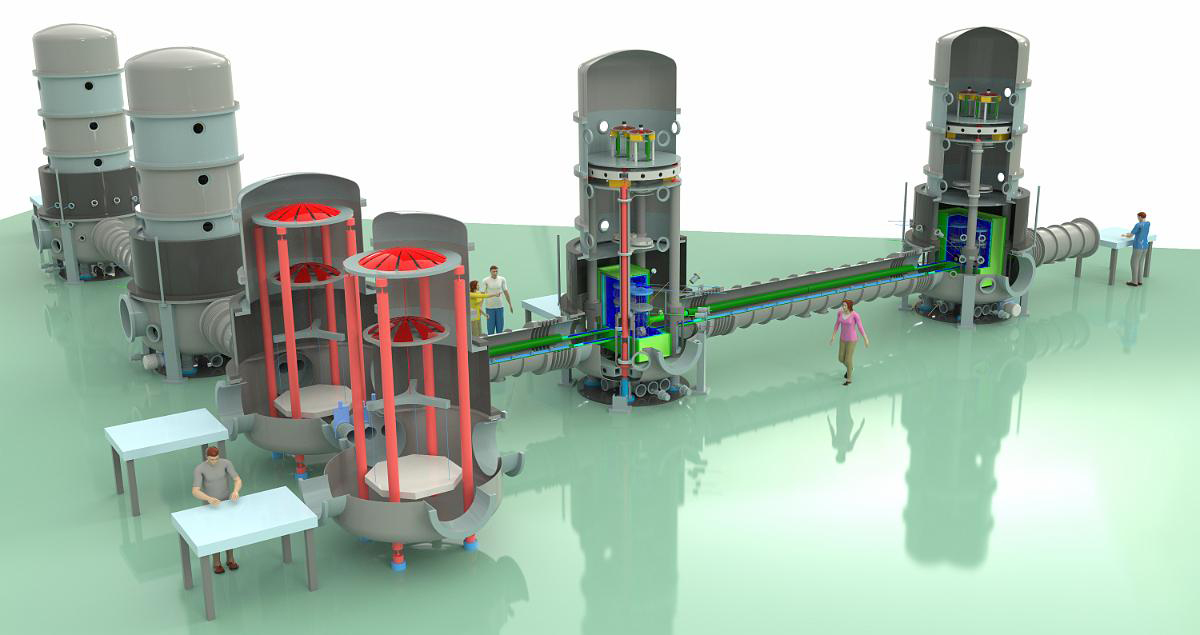
The ETpathfinder is a one-of-a-kind test facility for technologies of next-generation gravitational-wave detectors and is located in the ‘zwarte doos’, a former newspaper building in Maastricht’s Randwyck area.
Many of these developments can only be fully tested in an ultra-low noise environment, the ETpathfinder. It is a cryogenic testing platform that provides a similar infrastructure to that of large-scale detectors, but shrunk down to an arm length of around 10m. At ETpathfinder, we will try out novel techniques, help with design decisions for future gravitational-wave detectors, and train scientists to successfully use and transfer these techniques into the next detectors ET and CE.
Many of these developments can only be fully tested in an ultra-low noise environment, the ETpathfinder. It is a cryogenic testing platform that provides a similar infrastructure to that of large-scale detectors, but shrunk down to an arm length of around 10m. At ETpathfinder, we will try out novel techniques, help with design decisions for future gravitational-wave detectors, and train scientists to successfully use and transfer these techniques into the next detectors ET and CE.
Optical coatings
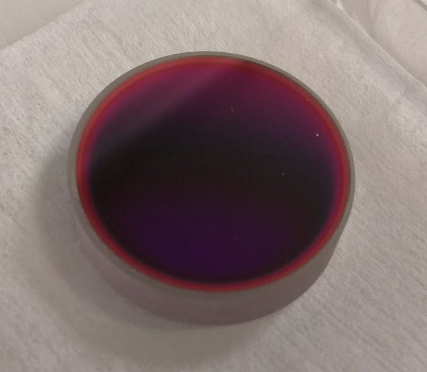
Advanced Virgo in Italy and Advanced LIGO in the US are currently the most sensitive gravitational-wave detectors in the world. To see more gravitational waves, originating from more distant or from different types of objects, the sensitivity of these highly-sensitive instruments needs to be further improved. We are working on different ways to improve the detectors, one of them being the highly-reflective optical coatings.
Thermal noise, caused by the highly-reflective mirror coatings, is one of the limiting factor in current gravitational-wave detectors. Thermal noise is determined by the material properties of the coatings and by their design and composition. However, other factors, such as extremely low optical absorption and scattering are also essential for these coatings.
A simultaneous optimization of all of these different aspects is challenging. Together with various collaborators we are working on coating deposition, on characterization and optimization of properties such as absorption and mechanical loss, on the optimization of the coating deposition procedure and of the coating design.
Quantum noise mitigation in GW detectors
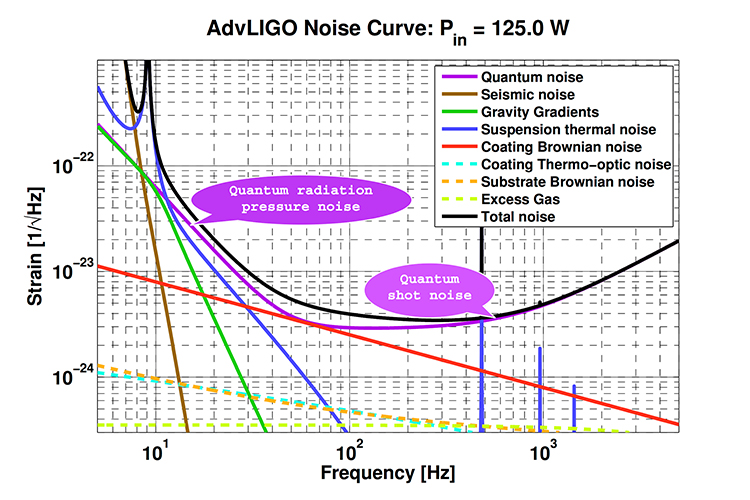
Current GW detectors, like Advanced LIGO and Advanced Virgo have reached an unprecedented level of sensitivity, at which fundamental vacuum fluctuations of light become the main limiting factor to further improvement. In order to reach its sensitivity goals, Einstein Telescope likewise the other next generation GW detectors will need to use advanced quantum measurement schemes to mitigate quantum noise.
Quantum noise originates from the quantum fluctuations of light amplitude and phase that follow the Heisenberg uncertainty relation. Quantum phase fluctuations can be viewed as a manifestation of Poissonian statistics of the photons emitted by an ideal laser and thus limit the measurement precision of any interferometric device. This uncertainty of photons arrival time at the photodiode known also as shot noise scales down with the increase of the number of photons used for the measurement and thus is inversely proportional to the light power interacting with the test masses of the interferometer. Amplitude fluctuations of light lead to the fluctuations of radiation pressure on the mirrors, resulting in the random motion of the mirrors and of the arm length change that mimics the gravitational wave signal. This second component of quantum noise is known as quantum radiation-pressure or quantum back-action noise. It naturally scales up with light power and is most prominent at the low frequencies, where massive suspended mirrors have higher response to the driving force. The fact that quantum shot noise and then quantum radiation-pressure noise have an inverse dependence on power and that the underlying fluctuations of phase and amplitude are uncorrelated, gives rise to a so called standard quantum limit (SQL) on continuous high-precision interferometric measurements.
In GWFP, we develop novel schemes of interferometers that are designed to circumvent the limitations of SQL and should become the base design for the next generation GW detectors.